Sleep, Light, Architecture: The Importance of Light for Human Circadian Rhythms
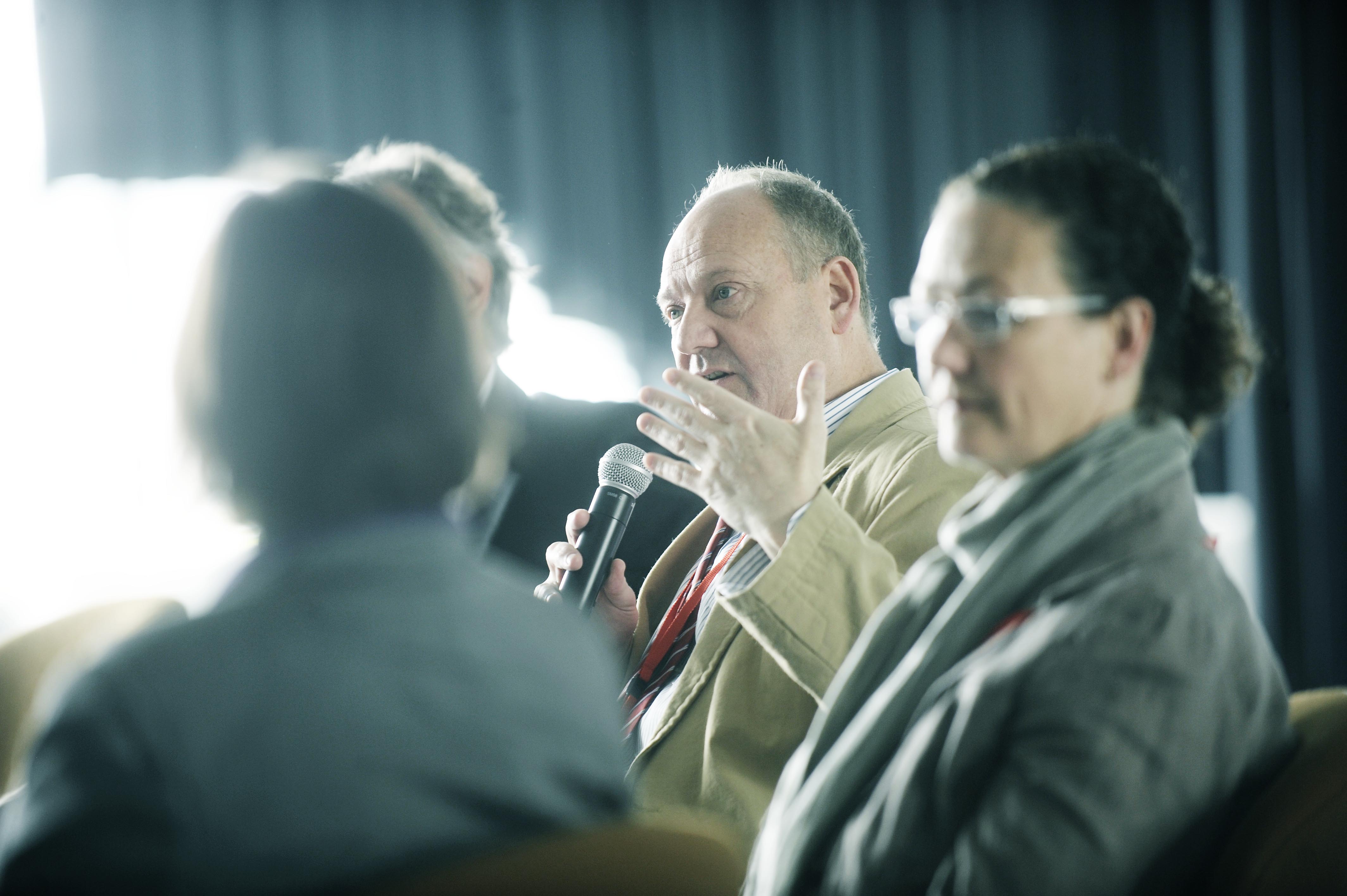
Category
Daylight
Health and Well-being
Author
Professor Russell G. Foster CBE, FRSB, FMedSci, FRS
Date
January 2023
Share
Copy
Russell Foster is Professor of Circadian Neuroscience, Director of the Sleep and Circadian Neuroscience Institute (SCNi) and Head of the Nuffield Laboratory of Ophthalmology at the University of Oxford. In 2015, he was honoured with a Commander of the Order of the British Empire (CBE) for services to science. In 2020, Russell Foster received The Daylight Award for Daylight research, presented by the VELUX Foundations and VELUX Stiftung.
Shedding Light on the Clock
One very striking feature of circadian rhythms, across all life, is that they do not run at exactly 24 hours and tick a little faster or a little slower. The human circadian system, on average, is 10 minutes longer than 24 hours, so without daily resetting we would get up and go to bed later by this amount each day. In this way, circadian rhythms resemble an old mechanical grandfather clock which needs a slight daily adjustment to make sure the clock is set to the “real” astronomical day. The most important signal that aligns (entrains) the internal day to the external world is light, and especially light at sunrise and sunset. The process of daily resetting is called “photoentrainment”. In us, and other mammals, eye loss prevents photoentrainment, and individuals who have lost their eyes, or are exposed to insufficient light, drift through time and experience something similar to unremitting jet lag.
The Features of Circadian Light Detection
In 1800, most of human society in Europe and the USA worked outside and was exposed to the natural cycle of light and dark. Today, in the UK, the proportion of workers in agriculture and fishing is 1% of the working population. This illustrates how the vast majority of the workforce in developed and developing nations has become profoundly detached from environmental light. And as a result, we have greatly diminished one of the most important signals that regulates our circadian rhythms and sleep/wake cycle.
With the benefit of hindsight, it is today surprising that before the late 1980’s human circadian rhythms were thought to be primarily regulated (entrained) by social cues, with little if any role for light. The first robust demonstration of light entrainment in humans used a Light/Dark (L/D) cycle of 5,000 lux. Levels of light that would be encountered outside – but not within buildings. We need to experience bright light, of the levels experienced outside, to robustly regulate our clocks (photoentrainment), and as illustrated in Figure 1, the levels of artificial light in our work and domestic spaces is often far below the levels of environmental light.
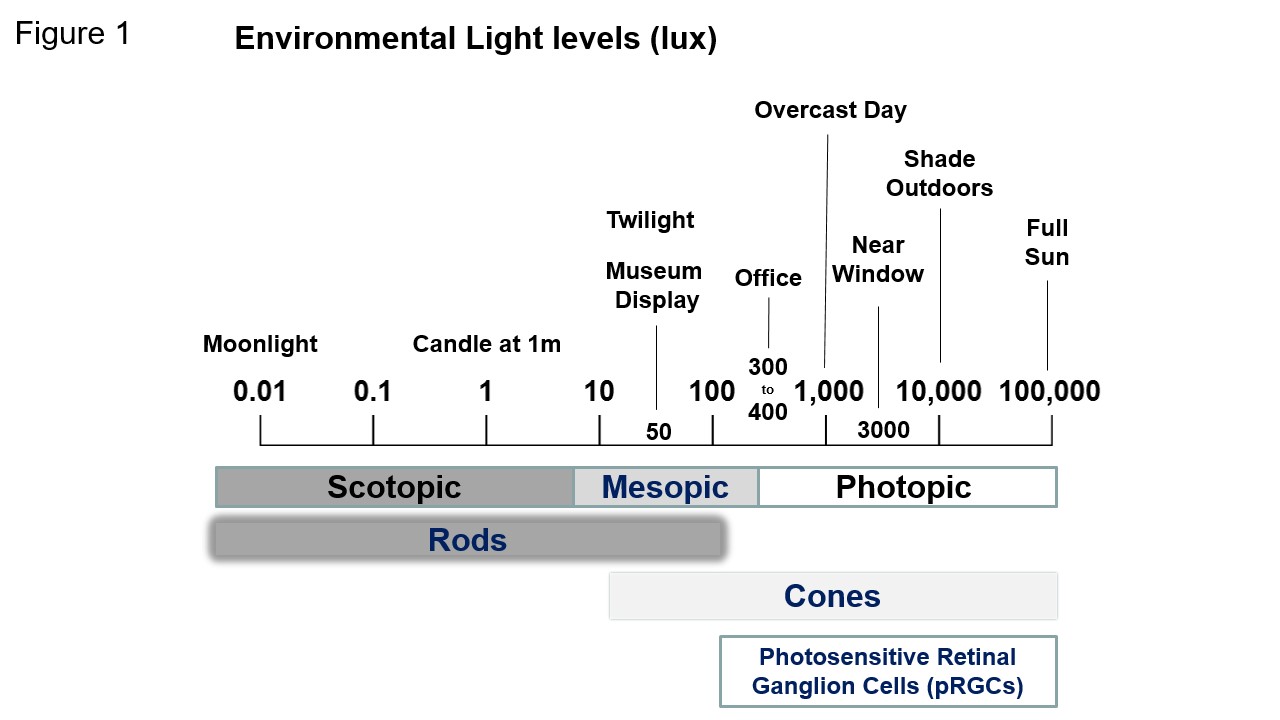
Environmental light levels expressed in lux. The human retina functions over a very wide range of light intensities. Encompassing Scotopic (dim light), Mesopic (twilight), and Photopic (daylight or other bright light) vision using rods, cones and a combination of both rods and cones. Light detection by the recently discovered melanopsin (OPN4)-based photosensitive retinal ganglion cells (pRGCs) requires relatively much brighter light. This diagram gives some sense of the sensory thresholds for the different photoreceptors. However, it is misleading because it fails to consider the differences in stimulus durations. Rods and cones detect light over the millisecond range whilst melanopsin pRGCs require long duration exposure (minutes to hours) to drive a response. The pRGCs can detect light in the 100 lux range for photoentrainment but it requires 4-6 hours of light exposure. An additional complication is that the rods and cones can modulate the light responses of the pRGCs (see below). The details are still emerging but the consensus is that rods may be important for photoentrainment at scotopic light intensities – under some circumstances; the rod and cone photoreceptors act together to contribute to photoentrainment at mesopic intensities, and the cones contribute at photopic levels of light, with the additional ability to integrate sudden changes in light intensity. The pRGCs detect light at relatively high irradiances and integrate light information over extended periods of time, and provide the main receptor for environmental twilight detection.
But it is not just light intensity that is important. The time of day we are exposed to light is critical. In brief, light approaching dusk in the early evening will delay the circadian clock and make us go to bed and get up later the next day. By contrast, early morning light will have the opposite effect, advancing the clock, making us go to bed and get up earlier. Light in the middle of the day is less effective, and some would say has little or no effect, in shifting the clock. This emphasizes the point that it is light exposure in the late afternoon and morning that is so important for photoentrainment. Yet, for urban humans, dawn vs dusk exposure can be very asymmetrical. Our recent study on university students from around the world, showed that the late types (“owls”) were exposed to light in the late afternoon (delaying light) but experienced little light in the morning (advancing light). The net effect was to shift the body clock to a later time. Another study from the USA examined sleep/wake timing in individuals after one week of maintaining daily routines of work, school, social activities, and exposure to electrical-lighting compared to one week of outdoor camping in tents and exposed to natural light. After the week of exposure to natural lighting, circadian timing and sleep/wake cycles had advanced by two hours! These two examples highlight the importance of taking both the intensity and timing of light into consideration when designing artificial lighting systems.
And what about the colour or wavelength of light? We demonstrated that there is a “3rd” photoreceptor within the eye, different from the rods and cones (Figure 2). These new photoreceptors are called “photosensitive retinal ganglion cells” (pRGCs) and form a photosensitive net within the eye, capturing light from all directions in space to provide an overall measure of light (irradiance/illuminance) within the environment to regulate the circadian system. The pRGCs utilize the “blue” light sensitive photopigment melanopsin (OPN4) which peaks in many species, including humans, close to 480nm.
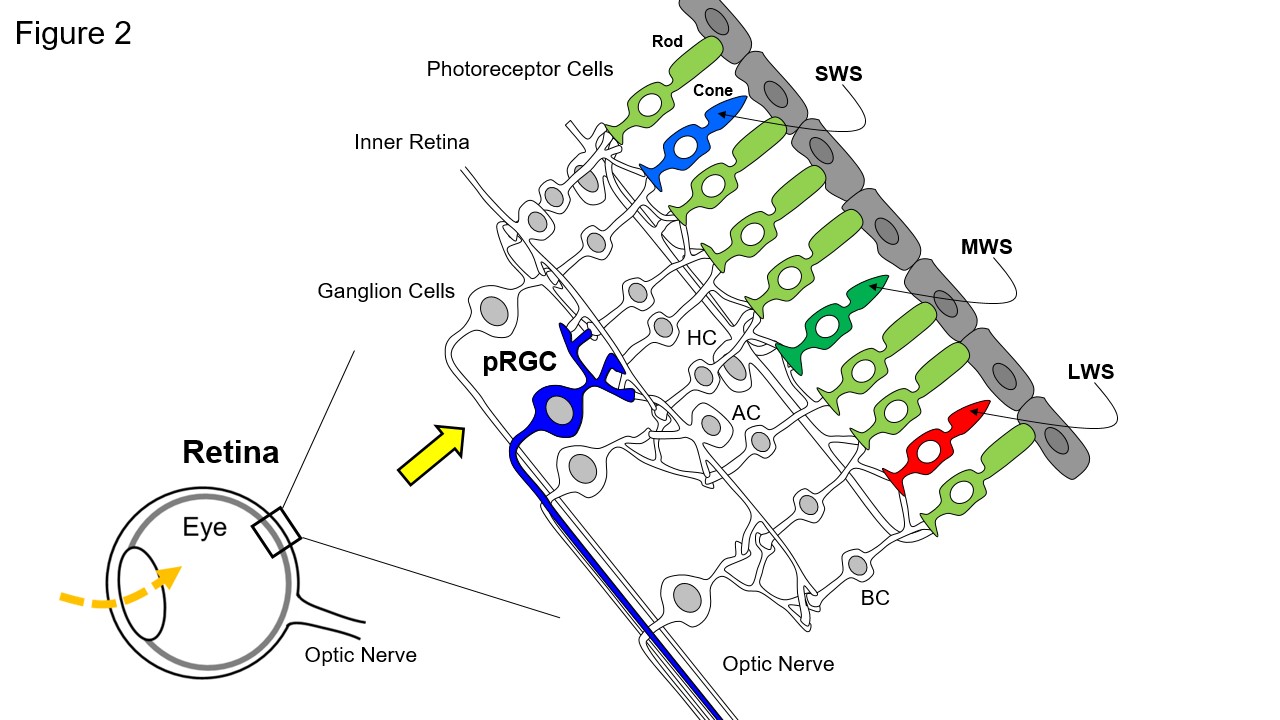
Diagram of the Human Retina. The rods and cones have differing spectral maxima (λmax): rod photoreceptors (Rod) [pale green], λmax ~ 498nm; Short wavelength sensitive (SWS) cones [pale blue], λmax ~ 420nm; Medium wavelength sensitive (MWS) cones [dark green], λmax ~ 534nm; Long wavelength sensitive (LWS) cones [red], λmax ~ 564nm. These photoreceptors convey visual information to the retinal ganglion cells via the second order neurones of the inner retina comprising the bipolar (BC), horizontal (HC) and amacrine (AC) cells. The optic nerve is formed from the axons of all the ganglion cells and this large nerve takes light information into the brain. A subset of photosensitive retinal ganglion cells (pRGC) [dark blue] detects light directly by using the “blue” light sensitive photopigment called melanopsin or OPN4. Thus, photodetection in the retina occurs in three types of cell: the rods, cones and pRGCs. There is anatomical and physiological evidence that rods and cones communicate with the pRGCs via the intermediate neurons of the retina (BC and HC) and modify the endogenous light responses of the pRGC.
Although we demonstrated that the rods and cones are not required for photoentrainment, they do play a role, acting to modify the endogenous photosensitivity of the pRGCs. But precisely how they do this remains poorly understood. Nevertheless, such interactions provide the potential to extend the spectral range for photoentrainment across most of the visible spectrum – not just “blue light”. It gets even more complex as inputs from short wavelength sensitive cones, peaking around 420nm, may even inhibit pRGC activation. As a result, it remains unclear how “excitatory” (or inhibitory) any light source is in regulating the circadian system, and in the absence of empirical physiological data, the current models that attempt to calculate the effectiveness of different artificial light sources on shifting the clock can, at best, only provide a very crude approximation. This said, laboratory studies suggest that the “blue light sensitive” pRGCs provide the dominant light input to the clock, and for the regulation of alertness and mood, under extended lighting conditions.
The use of light-emitting devices immediately before bedtime has been a concern in some quarters because of the potential impact these devices may have on human circadian timing, not least because the light they emit is enriched in blue light. Indeed, in the popular press, the use of electronic devices before bedtime is “known” to alter our circadian rhythms. Whereas most studies have identified only small, if any, effects in response to such low levels of light. The most detailed study to-date compared reading a Light-Emitting (LE)-eBook in dim room light for ∼4 h (18:00 – 22:00) before bedtime for five consecutive evenings vs reading a printed book under the same conditions. The light emitted from the LE-eBook was ~ 31 lux whilst the light reflected from the printed book was ~ 1 lux. Both these intensities would have been easily detected by rod photoreceptors. The results showed that LE e-Book use delayed sleep onset by less than 10 minutes after five consecutive days of exposure, compared to reading the printed book. Although the results were statistically significant (just), a delay of 10 min. is almost meaningless. These data suggest that under normal circumstances light-emitting screens are not sufficiently bright to shift the circadian system, and that rod photoreceptors, which would easily detect such low levels of light, are not feeding this information to the pRGCs for photoentrainment.
What about changing the screen colour of a computer? A cross-platform computer program has been developed called “f.lux” that adjusts a computer screens colour temperature according to location and time of day based upon local sunrise and sunset. The effect is to reduce the irradiance and blue light emission from a screen in the evening compared to the morning. The proponents of f.lux hypothesise that altering the color temperature of the display to reduce the prominence of blue light at night will improve the sleep and reduce circadian rhythm disruption. Although the developer provides a list of relevant research on the website (justgetflux.com), the program itself has not been tested to determine its efficacy. In spite of this, f.lux has been widely and positively reviewed by technology journalists, bloggers, and users.
Whilst it is probably sensible to minimise light exposure prior to bedtime to reduce levels of alertness, which has a lower threshold than photoentrainment, the impact of light from digital devices on circadian entrainment appears small at best. But this does not mean we should use devices before bedtime. Studies have shown that late night use of computer games, social media, or smart-phone increase brain alertness which can in turn greatly delay and disrupt sleep. As a result, such devices should not be used 30 minutes prior to bedtime, and if you wake in the night, do not be tempted to abandon sleep and use these devices. Sleep is likely to return if you remain sufficiently relaxed.
The Ecology of Human Light Exposure
There is clearly a complex relationship between light duration, intensity, and wavelength for photoentrainment. Furthermore, light exposure at dusk will delay the circadian system (go to bed and get up later), whilst early evening light exposure will advance the circadian system (go to bed and get up earlier). There is also evidence that an individual’s “light history” or prior light exposure to light (long-term working inside vs outside) will modify circadian entrainment. And finally, an individual’s age, the rate of change of light intensity experienced at twilight (rapid vs slow light transitions) and the seasons will all act to modulate photoentrainment. These key factors, along with ill-defined photoreceptors interactions between pRGCs, rods and cones, illustrate the fact that attempts to develop evidence-based human centric lighting to enhance the effects of artificial light on the circadian system (or reduce it) in our built spaces is not straightforward. Trying to gather the missing information for such modelling based upon laboratory studies would take both time and require very significant resources. Perhaps the way forward is to measure how humans respond to dynamic light exposure in the “real world” were light intensity, duration, spectral quality and the time of exposure vary greatly as we move back and forth between buildings and the outside. Such an approach is now feasible because devices are available that measure non-invasively, continuously and over an extended period of time (weeks) light exposure (irradiance, duration, wavelength, time of exposure) and individual circadian timing (sleep/wake, and other measures of circadian timing), alertness and mood. Such measurements, in relatively large numbers of individuals, across a diverse demographic, would provide a means to identify how key features of natural light exposure interact to achieve entrainment (or not), along with enhanced mood and alertness. I am strongly of the view that such natural light experiments will provide key information regarding our understanding of those features of the light environment that are important for photoentrainment and wellbeing – and by extension – what is required for the development of truly innovative artificial human centric lighting in our buildings of the future. But I stress, this current lack of specific knowledge should not be an excuse to ignore the importance of light in our built spaces.
Implications for Architecture
Although we do not currently have a complete understanding of how the human circadian system is regulated by light, we do know that light exposure, of the sort encountered within the natural environment, provides the optimum set of signals for photoentrainment, alertness and mood. As a result, it is of overwhelming importance that architects find ways to maximize the penetration of natural light into our built spaces. A buildings aesthetics and utility are, of course, critical. But as a first principle, these considerations should never eclipse the use of natural light to illuminate our civic, domestic and commercial spaces. The idea that a building can be designed, and then as a secondary consideration, be illuminated artificially by employing ever-more complex lighting systems is, in my view, both lazy and a dereliction of responsibility. There will be circumstances where artificial light is the only option, and we should certainly strive to define how to optimize such lighting systems as a “last resort”. But my hope is that a hallmark of 21st century architecture will be to truly embrace the possibilities of natural light, and that every project will start by prioritising how natural light can be used to achieve high quality and healthy spaces to enhance human performance.
Further Reading
Foster, R. G., S. Hughes and S. N. Peirson (2020). “Circadian Photoentrainment in Mice and Humans.” Biology (Basel) 9(7).
Russell Foster. Life Time: The new science of the body clock and how it can revolutionise your sleep and health. Penguin Books UK; Yale University Press USA, (2022)